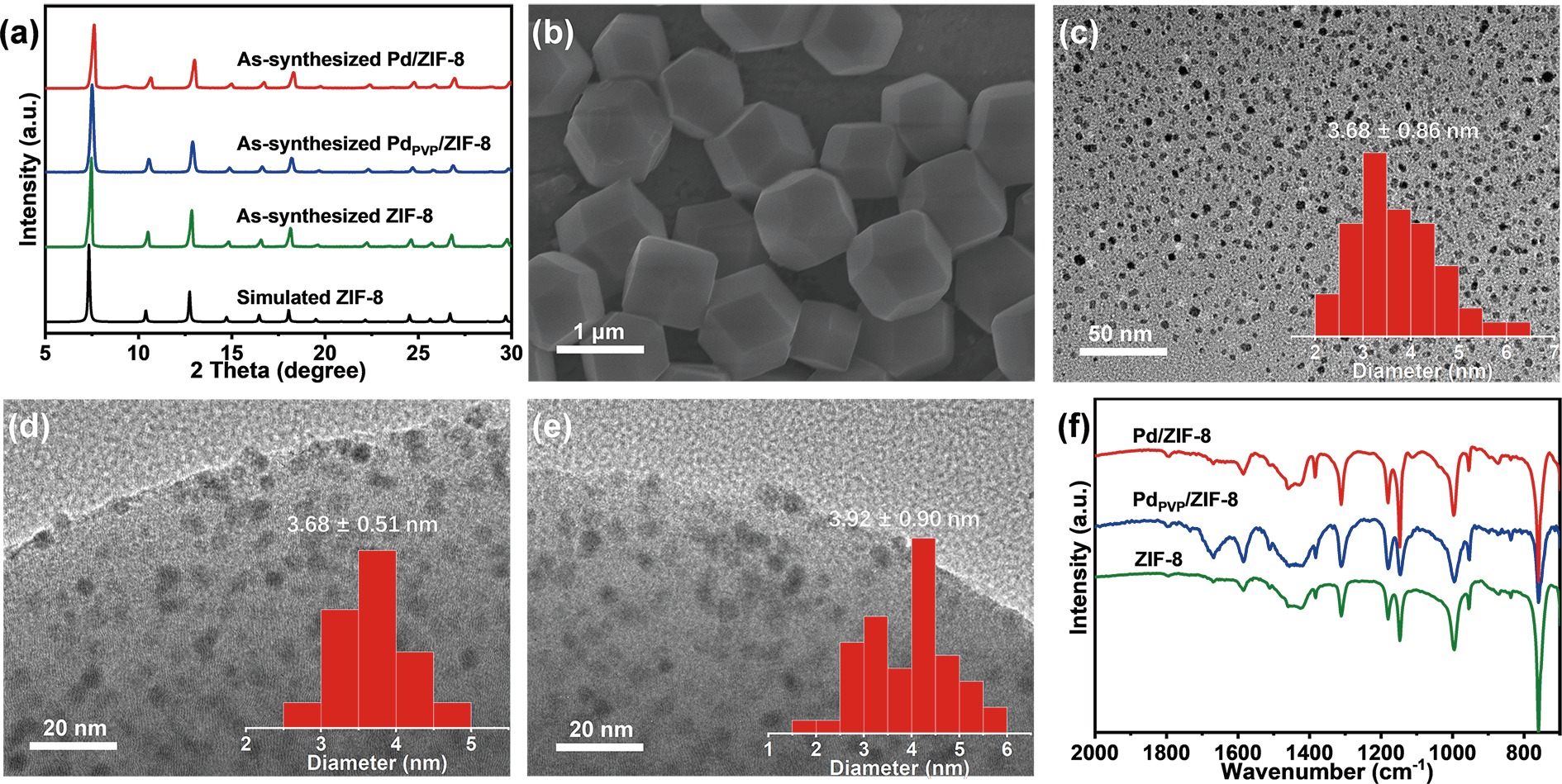
Palladium-catalyzed C-C coupling reactions are of significant importance, but they often require harsh conditions. Herein, we report an interface-regulated photocatalytic Suzuki coupling reaction over Pd nanoparticles supported on a metal-organic framework (MOF), ZIF-8. Two Pd/MOFs were synthesized, PdPVP/ZIF-8 and Pd/ZIF-8, which have similar Pd sizes and loading amounts, except that the former contains poly(vinylpyrrolidone) (PVP) as a surfactant. The diffuse-reflectance infrared Fourier transform of CO adsorption (CO-DRIFT) indicates that Pd/ZIF-8 represents a more negative electronic state of Pd than PdPVP/ZIF-8. In the photocatalytic Suzuki coupling reaction between iodobenzene and phenylboronic acid, Pd/ZIF-8 exhibits excellent performance (99.1% yield), much better than that of PdPVP/ZIF-8 (57.9% yield). Moreover, Pd/ZIF-8 is highly stable and shows broad substrate scope for this reaction. The superior activity of Pd/ZIF-8 can be attributed to sufficient electron transfer between the MOFs and Pd nanoparticles in the absence of an interfacial surfactant. This work provides new insights into a Pd-catalyzed C-C coupling reaction involving photocatalysis and interfacial electron transfer.
Interfacial electron transfer determines the Pd photocatalytic activity in Pd/ZIF-8.
Figure 1. (a) Powder XRD patterns of simulated ZIF-8 and as-synthesized ZIF-8, PdPVP/ZIF-8, and Pd/ZIF-8. (b) SEM image of ZIF-8. (c–e) TEM images for (c) PdPVP nanoparticles, (d) PdPVP/ZIF-8, and (e) Pd/ZIF-8 . Insets are the size distributions of Pd nanoparticles. (f) IR spectra of ZIF-8, PdPVP/ZIF-8, and Pd/ZIF-8.
Figure 3. (a) Time-dependent yields of biphenyl in reactions of PdPVP/ZIF-8 and Pd/ZIF-8 (the error bars represent the relative deviation obtained from parallel experiments). (b, c) Recycling performance of (b) Pd/ZIF-8 and (c) PdPVP/ZIF-8. (d) Comparison of powder XRD patterns of PdPVP/ZIF-8 and Pd/ZIF-8 before and after reaction. (e, f) TEM images of (e) PdPVP/ZIF-8 and (f) Pd/ZIF-8 after reaction (inset: size distribution of Pd nanoparticles).
[1] |
Miyaura N, Suzuki, A. Stereoselective synthesis of arylated (E) -alkenes by the reaction of alk-1 -enylboranes with aryl halides in the presence of palladium catalyst. J. C. S. Chem. Comm., 1979: 866–867. DOI: 10.1039/C39790000866
|
[2] |
Miyaura N, Yamada K, Suzuki A. A new stereospecific cross-coupling by the palladium-catalyzed reaction of 1-alkenylboranes with 1-alkenyl or 1-alkynyl halides. Tetrahedron Lett., 1979, 20 (36): 3437–3440. DOI: 10.1016/S0040-4039(01)95429-2
|
[3] |
Buchwald S L. Cross coupling. Acc. Chem. Res., 2008, 41 (11): 1439. DOI: 10.1021/ar8001798
|
[4] |
Wang F, Mielby J, Richter F H, et al. A polyphenylene support for Pd catalysts with exceptional catalytic activity. Angew. Chem. Int. Ed., 2014, 53 (33): 8645–8648. DOI: 10.1002/anie.201404912
|
[5] |
Sarina S, Zhu H Y, Xiao Q, et al. Viable photocatalysts under solar-spectrum irradiation: nonplasmonic metal nanoparticles. Angew. Chem. Int. Ed., 2014, 53 (11): 2935–2940. DOI: 10.1002/anie.201308145
|
[6] |
Jin Z, Xiao M D, Bao Z H, et al. A general approach to mesoporous metal oxide microspheres loaded with noble metal nanoparticles. Angew. Chem. Int. Ed., 2012, 51 (26): 6406–6410. DOI: 10.1002/anie.201106948
|
[7] |
Wang Y Q, Wang, C T, Wang L X, et al. Zeolite fixed metal nanoparticles: New perspective in catalysis. Acc. Chem. Res., 2021, 54 (11): 2579–2590. DOI: 10.1021/acs.accounts.1c00074
|
[8] |
Li X H, Antonietti M. Metal nanoparticles at mesoporous N-doped carbons and carbon nitrides: functional Mott-Schottky heterojunctions for catalysis. Chem. Soc. Rev., 2013, 42 (16): 6593–6604. DOI: 10.1039/C3CS60067J
|
[9] |
van Deelen T W, Hernández Mejía C, de Jong K P. Control of metal-support interactions in heterogeneous catalysts to enhance activity and selectivity. Nat. Catal., 2019, 2 (11): 955–970. DOI: 10.1038/s41929-019-0364-x
|
[10] |
Nilsson Pingel T, Jørgensen M, Yankovich A B, et al. Influence of atomic site-specific strain on catalytic activity of supported nanoparticles. Nat. Commun., 2018, 9 (1): 2722. DOI: 10.1038/s41467-018-05055-1
|
[11] |
Huang J, He S, Goodsell J L, et al. Manipulating atomic structures at the Au/TiO2 interface for O2 activation. J. Am. Chem. Soc., 2020, 142 (14): 6456–6460. DOI: 10.1021/jacs.9b13453
|
[12] |
Komanoya T, Kinemura T, Kita Y, et al. Electronic effect of ruthenium nanoparticles on efficient reductive amination of carbonyl compounds. J. Am. Chem. Soc., 2017, 139 (33): 11493–11499. DOI: 10.1021/jacs.7b04481
|
[13] |
Wang H, Wang L, Lin D, et al. Strong metal-support interactions on gold nanoparticle catalysts achieved through Le Chatelier’s principle. Nat. Catal., 2021, 4 (5): 418–424. DOI: 10.1038/s41929-021-00611-3
|
[14] |
Furukawa H, Cordova K E, O'Keeffe M, et al. The chemistry and applications of metal-organic frameworks. Science, 2013, 341 (6149): 1230444. DOI: 10.1126/science.1230444
|
[15] |
Zhou H C, Kitagawa S. Metal-organic frameworks (MOFs). Chem. Soc. Rev., 2014, 43 (16): 5415–5418. DOI: 10.1039/C4CS90059F
|
[16] |
Li H, Li L B, Lin R B, et al. Porous metal-organic frameworks for gas storage and separation: Status and challenges. EnergyChem, 2019, 1 (1): 100006. DOI: 10.1016/j.enchem.2019.100006
|
[17] |
Yang Q H, Xu Q, Jiang H L. Metal-organic frameworks meet metal nanoparticles: synergistic effect for enhanced catalysis. Chem. Soc. Rev., 2017, 46 (15): 4774–4808. DOI: 10.1039/C6CS00724D
|
[18] |
Li L Y, Li Z X, Yang W J, et al. Integration of Pd nanoparticles with engineered pore walls in MOFs for enhanced catalysis. Chem, 2021, 7 (3): 686–698. DOI: 10.1016/j.chempr.2020.11.023
|
[19] |
Kolobov N, Goesten M G, Gascon J. Metal-organic frameworks: molecules or semiconductors in photocatalysis? Angew. Chem. Int. Ed., 2021, 60 (50): 26038–26052. DOI: 10.1002/anie.202106342
|
[20] |
Xiao J D, Han L L, Luo J, et al. Integration of plasmonic effects and schottky junctions into metal-organic framework composites: steering charge flow for enhanced visible-light photocatalysis. Angew. Chem. Int. Ed., 2018, 57 (4): 1103–1107. DOI: 10.1002/anie.201711725
|
[21] |
Xu M L, Li D D, Sun K, et al. Interfacial microenvironment modulation boosting electron transfer between metal nanoparticles and MOFs for enhanced photocatalysis. Angew. Chem. Int. Ed., 2021, 60 (30): 16372–16376. DOI: 10.1002/anie.202104219
|
[22] |
Park K S, Ni Z, Côté A P, et al. Exceptional chemical and thermal stability of zeolitic imidazolate frameworks. Proc. Natl. Acad. Sci. U. S. A., 2006, 103: 10186–10191. DOI: 10.1073/pnas.0602439103
|
[23] |
Huang X C, Lin Y Y, Zhang J P, et al. Ligand-directed strategy for zeolite-type metal-organic frameworks: zinc(II) imidazolates with unusual zeolitic topologies. Angew. Chem. Int. Ed., 2006, 45 (10): 1557–1559. DOI: 10.1002/anie.200503778
|
[24] |
Li L Y, Yang W J, Yang Q H, et al. Accelerating chemo- and regioselective hydrogenation of alkynes over bimetallic nanoparticles in a metal–organic framework. ACS Catal., 2020, 10 (14): 7753–7762. DOI: 10.1021/acscatal.0c00177
|
[25] |
Xu H Q, Hu J H, Wang D K, et al. Visible-light photoreduction of CO2 in a metal-organic framework: boosting electron-hole separation via electron trap states. J. Am. Chem. Soc., 2015, 137 (42): 13440–13443. DOI: 10.1021/jacs.5b08773
|
[26] |
Pei L, Li T Z, Yuan Y J, et al. Schottky junction effect enhanced plasmonic photocatalysis by TaON@Ni NP heterostructures. Chem. Commun., 2019, 55 (78): 11754–11757. DOI: 10.1039/C9CC05485E
|
[27] |
Lo W S, Chou L Y, Young A P, et al. Probing the interface between encapsulated nanoparticles and metal-organic frameworks for catalytic selectivity control. Chem. Mater., 2021, 33 (6): 1946–1953. DOI: 10.1021/acs.chemmater.0c03007
|
[28] |
Hayyan M, Hashim M A, AlNashef I M. Superoxide ion: Generation and chemical implications. Chem. Rev., 2016, 116 (5): 3029–3085. DOI: 10.1021/acs.chemrev.5b00407
|
[29] |
htarev D S, Shtareva A V, Blokh A I, et al. On the question of the optimal concentration of benzoquinone when it is used as a radical scavenger. Appl. Phys. A, 2017, 123 (9): 602. DOI: 10.1007/s00339-017-1193-x
|
[30] |
Luo S Q, Ren X H, Lin H W, et al. Plasmonic photothermal catalysis for solar-to-fuel conversion: current status and prospects. Chem. Sci, 2021, 12 (16): 5701–5719. DOI: 10.1039/d1sc00064k
|
[31] |
Prajapati P K, Saini S, Jain S L. Nickel mediated palladium free photocatalytic Suzuki-coupling reaction under visible light irradiation. J. Mater. Chem. A, 2020, 8 (10): 5246–5254. DOI: 10.1039/C9TA13801C
|
[32] |
Wang Z J, Ghasimi S, Landfester K, et al. Photocatalytic Suzuki coupling reaction using conjugated microporous polymer with immobilized palladium nanoparticles under visible light. Chem. Mater., 2015, 27 (6): 1921–1924. DOI: 10.1021/acs.chemmater.5b00516
|
[33] |
MacQuarrie S, Horton J H, Barnes J, et al. Visual observation of redistribution and dissolution of palladium during the Suzuki-Miyaura reaction. Angew. Chem. Int. Ed., 2008, 47 (17): 3279–3282. DOI: 10.1002/anie.200800153
|
[34] |
Bai S, Jiang J, Zhang Q, et al. Steering charge kinetics in photocatalysis: intersection of materials syntheses, characterization techniques and theoretical simulations. Chem. Soc. Rev., 2015, 44: 2893–2939. DOI: 10.1039/C5CS00064E
|
[35] |
Avci C, Imaz I, Carne-Sanchez A, et al. Self-assembly of polyhedral metal-organic framework particles into three-dimensional ordered superstructures. Nat. Chem., 2017, 10 (1): 78–84. DOI: 10.1038/nchem.2875
|
[36] |
Xiao J D, Shang Q C, Xiong Y J, et al. Boosting photocatalytic hydrogen production of a metal–organic framework decorated with platinum nanoparticles: The platinum location matters. Angew. Chem. Int. Ed., 2016, 55: 9389–9393. DOI: 10.1002/anie.201603990
|
[37] |
Liu H, Xu C Y, Li D D, et al. Photocatalytic hydrogen production coupled with selective benzylamine oxidation over MOF composites. Angew. Chem. Int. Ed., 2018, 57 (19): 5379–5383. DOI: 10.1002/anie.201800320
|
Catalyst | Contenta |
PdPVP/ZIF-8 | 2.01 wt% |
Pd/ZIF-8 | 1.98 wt% |
PdPVP/ZIF-8 after reaction | 1.99 wt% |
Pd/ZIF-8 after reaction | 1.96 wt% |
[Note] a The Pd contents were confirmed by ICP-AES data. |
Entry | Substrate 1 | Substrate 2 | Conversion of 1 (%) | Yield (%) |
1 | ![]() | ![]() | 38.60 | 38.42 |
2 | ![]() | ![]() | 0.06 | 0.06 |
3 | ![]() | ![]() | 99.99 | 99.92 |
4 | ![]() | ![]() | 99.99 | 99.25 |
5 | ![]() | ![]() | 96.52 | 96.13 |
6 | ![]() | ![]() | 99.85 | 99.69 |
[Note] a Standard conditions: typically, 10 mg catalyst, 80 mg K2CO3, 10 μL iodobenzene (0.1 mmol), 24 mg phenylboronic acid (0.2 mmol) and 2 mL DMF/water (1/1, v/v), LED lamp (450 nm, 80 W), 5 h. The conversion and yield were determined by GC analysis, and n-dodecane was used as the internal standard. |
Entry | Catalyst | Base | Light | Atmosphere | Additive | Temperature (oC) | Yield (%) |
1 | Pd/ZIF-8 | − | + | N2 | − | 25 | n.d. |
2 | Pd/ZIF-8 | + | − | N2 | − | 25 | n.d. |
3 | Pd/ZIF-8 | + | − | N2 | − | 60 | 34.32 |
4 | Pd/ZIF-8 | + | + | O2 | − | 25 | n.d. |
5 | Pd/ZIF-8 | + | + | N2 | pBQ | 25 | 70.56 |
6 | Pd/ZIF-8 | + | + | N2 | MeOH | 25 | 67.15 |
7 | ZIF-8 | + | + | N2 | − | 25 | n.d. |
8b | PdPVP+ ZIF-8 | + | + | N2 | − | 25 | 38.42 |
[Note] a Standard conditions: typically, 10 mg catalyst, 80 mg K2CO3, 10 μL iodobenzene (0.1 mmol), 24 mg phenylboronic acid (0.2 mmol) and 2 mL DMF/water (1/1, v/v), LED lamp (450 nm, 80 W), 5 h. The conversion and yield were determined by GC analysis, and n-dodecane was used as the internal standard. b 100 μL PdPVP aqueous solution and 10 mg ZIF-8 were added to 1mL of DMF and 0.9 mL deionized water while the other experimental parameters were maintained constant. |