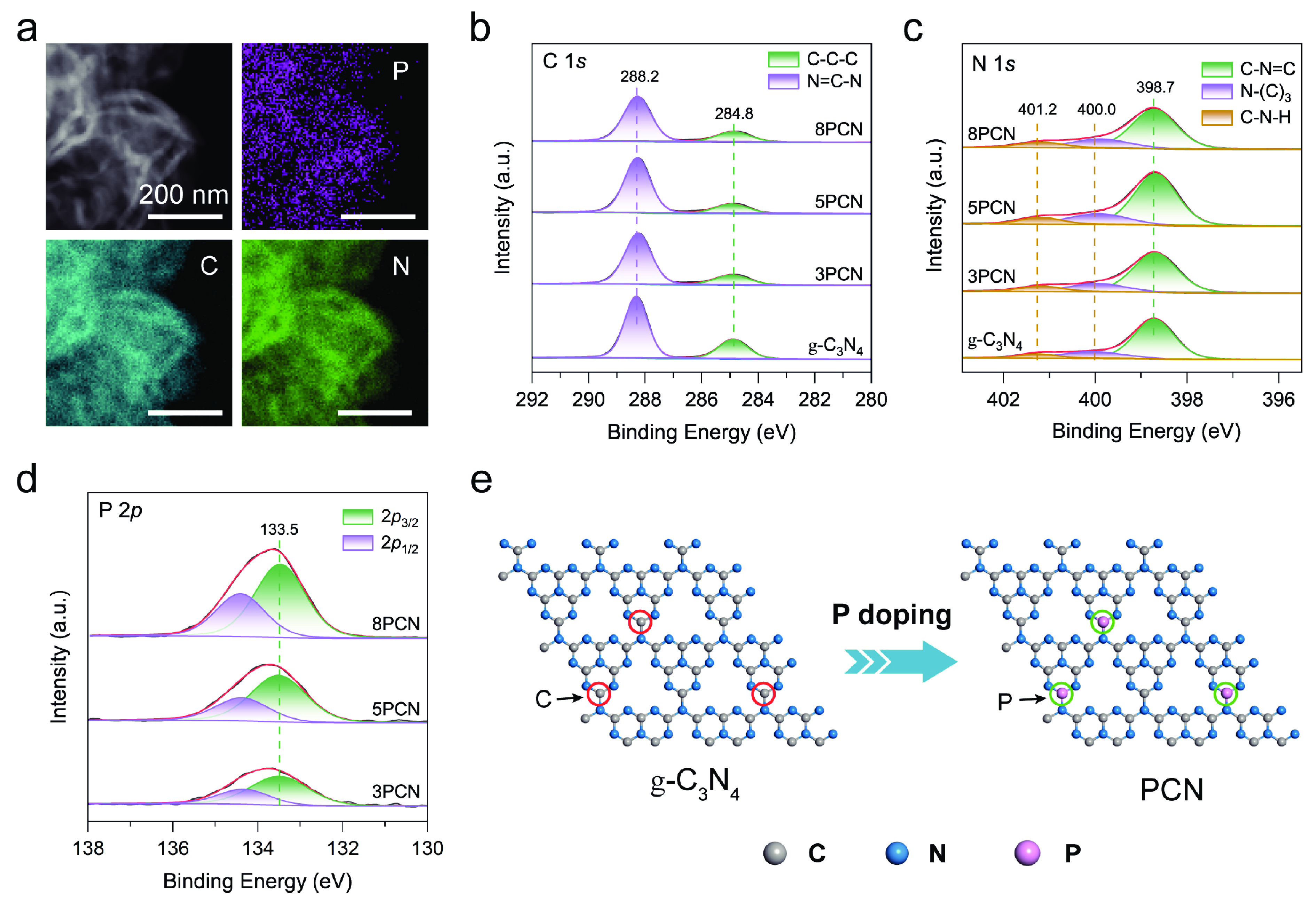
By simplifying catalyst–product separation and reducing phosphorus waste, heterogeneous hydroformylation offers a more sustainable alternative to homogeneous processes. However, heterogeneous hydroformylation catalysts developed thus far still suffer from the issues of much lower activity and metal leaching, which severely hinder their practical application. Here, we demonstrate that incorporating phosphorus (P) atoms into graphitic carbon nitride (PCN) supports facilitates charge transfer from Rh to the PCN support, thus largely enhancing electronic metal–support interactions (EMSIs). In the styrene hydroformylation reaction, the activity of Rh1/PCN single-atom catalysts (SACs) with varying P contents exhibited a volcano-shaped relationship with P doping, where the Rh1/PCN SAC with optimal P doping showed exceptional activity, approximately 5.8- and 3.3-fold greater than that of the Rh1/g-C3N4 SAC without P doping and the industrial homogeneous catalyst HRh(CO)(PPh3)3, respectively. In addition, the optimal Rh1/PCN SAC catalyst also demonstrated largely enhanced multicycle stability without any visible metal aggregation owing to the increased EMSIs, which sharply differed from the severe metal aggregation of large nanoparticles on the Rh1/g-C3N4 SAC. Mechanistic studies revealed that the enhanced catalytic performance could be attributed to electron-deficient Rh species, which reduced CO adsorption while simultaneously promoting alkene adsorption through increased EMSIs. These findings suggest that tuning EMSIs is an effective way to achieve SACs with high activity and durability.
Phosphorus doping of carbon nitride-supported Rh single-atom catalysts markedly increases their activity and stability in the heterogeneous hydroformylation reaction.
Figure 1. Structural characterization of PCN. (a) A STEM image of 3PCN and corresponding EDS maps of P (rose red), C (cyan), and N (green). XPS spectra of g-C3N4, 3PCN, 5PCN and 8PCN in the C 1 s (b), N 1 s (c) and P 2p (d) regions. (e) Structure diagram of P-doped g-C3N4. The gray, blue, and carmine balls denote carbon, nitrogen and phosphorus atoms, respectively.
Figure 2. Structural characterization. Aberration-corrected HAADF-STEM images of Rh1/g-C3N4 at low (a) and high (b) magnifications, showing that Rh single atoms are present (marked by yellow circles). Aberration-corrected HAADF-STEM image of Rh1/5PCN at low (c) magnification. (d) Corresponding EDS maps of P (rose red), C (cyan), N (green) and Rh (yellow) for Rh1/5PCN. Aberration-corrected HAADF-STEM image of Rh1/5PCN at high (e) magnification. (f) In situ DRIFT spectra of CO chemisorption on the Rh1/g-C3N4, Rh1/3PCN, Rh1/5PCN and Rh1/8PCN catalysts. XPS spectra of Rh1/g-C3N4, Rh1/3PCN, Rh1/5PCN and Rh1/8PCN in the Rh 3d (g), N 1 s (h) and P 2p (i) regions.
Figure 3. Catalytic performance in styrene hydroformylation. Comparing the TOF (a) and selectivity (b) for Rh1/g-C3N4, Rh1/3PCN, Rh1/5PCN, Rh1/8PCN and HRh(CO)(PPh3)3 for the hydroformylation of styrene. The l/b ratio represents the molar ratio of 3-phenylpropanal to 2-phenylpropanal in the product mixture. Reaction conditions: 2 mmol of styrene, 10 mL of toluene, 30 bar of syngas, 100 °C. The TOF of each catalyst was calculated on the basis of the yield of aldehydes at a conversion level of < 20% using each catalyst. (c) Arrhenius plots and calculated apparent activation energies in the kinetic reaction regions. (d) Kinetic reaction order with respect to CO and styrene during the hydroformylation of styrene. Recyclability of Rh1/g-C3N4 (e) and Rh1/5PCN (f) for styrene hydroformylation.
Figure 4. Morphology of the Rh1/g-C3N4 and Rh1/5PCN samples after styrene hydroformylation stability testing. TEM images at low (a) and high (b, c) magnifications of the Rh1/g-C3N4 used after 4 cycles of the styrene hydroformylation test. The aggregated Rh nanoparticles in a-c are highlighted by red circles. Aberration-corrected HAADF-STEM images at low (d) and high (e, f) magnifications of Rh1/5PCN after 5 cycles of the styrene hydroformylation test; the Rh single atoms in e and f are highlighted by yellow circles.
[1] |
Franke R, Selent D, Börner A. Applied hydroformylation. Chemical Reviews, 2012, 112 (11): 5675–5732. DOI: 10.1021/cr3001803
|
[2] |
Peng J B, Geng H Q, Wu X F. The chemistry of CO: carbonylation. Chem, 2019, 5 (3): 526–552. DOI: 10.1016/j.chempr.2018.11.006
|
[3] |
Gao P, Liang G F, Ru T, et al. Phosphorus coordinated Rh single-atom sites on nanodiamond as highly regioselective catalyst for hydroformylation of olefins. Nature Communications, 2021, 12: 4698.
|
[4] |
Frey G D. 75 Years of oxo synthesis–The success story of a discovery at the OXEA Site Ruhrchemie. Journal of Organometallic Chemistry, 2014, 754: 5–7. DOI: 10.1016/j.jorganchem.2013.12.023
|
[5] |
Hood D M, Johnson R A, Carpenter A E, et al. Highly active cationic cobalt(II) hydroformylation catalysts. Science, 2020, 367 (6477): 542–548. DOI: 10.1126/science.aaw7742
|
[6] |
Zhang B, Kubis C, Franke R. Hydroformylation catalyzed by unmodified cobalt carbonyl under mild conditions. Science, 2022, 377 (6611): 1223–1227. DOI: 10.1126/science.abm4465
|
[7] |
Brezny A C, Landis C R. Recent developments in the scope, practicality, and mechanistic understanding of enantioselective hydroformylation. Accounts of Chemical Research, 2018, 51 (9): 2344–2354. DOI: 10.1021/acs.accounts.8b00335
|
[8] |
Li P, Kawi S. SBA-15-based polyamidoamine dendrimer tethered Wilkinson’s rhodium complex for hydroformylation of styrene. Journal of Catalysis, 2008, 257 (1): 23–31. DOI: 10.1016/j.jcat.2008.04.002
|
[9] |
Amsler J, Sarma B B, Agostini G, et al. Prospects of heterogeneous hydroformylation with supported single atom catalysts. Journal of the American Chemical Society, 2020, 142 (11): 5087–5096. DOI: 10.1021/jacs.9b12171
|
[10] |
Tan M H, Yang G H, Wang T J, et al. Active and regioselective rhodium catalyst supported on reduced graphene oxide for 1-hexene hydroformylation. Catalysis Science & Technology, 2016, 6 (4): 1162–1172.
|
[11] |
Shi Y K, Hu X J, Zhu B L, et al. Synthesis and characterization of TiO2 nanotube supported Rh-nanoparticle catalysts for regioselective hydroformylation of vinyl acetate. RSC Advances, 2014, 4 (107): 62215–62222. DOI: 10.1039/C4RA11156G
|
[12] |
Sordelli L, Guidotti M, Andreatta D, et al. Characterization and catalytic performances of alkali-metal promoted Rh/SiO2 catalysts for propene hydroformylation. Journal of Molecular Catalysis A: Chemical, 2003, 204: 509–518.
|
[13] |
Hou C, Zhao G, Ji Y, et al. Hydroformylation of alkenes over rhodium supported on the metal-organic framework ZIF-8. Nano Research, 2014, 7: 1364–1369. DOI: 10.1007/s12274-014-0501-4
|
[14] |
Liu B, Wang Y, Huang N, et al. Heterogeneous hydroformylation of alkenes by Rh-based catalysts. Chem, 2022, 8 (10): 2630–2658. DOI: 10.1016/j.chempr.2022.07.020
|
[15] |
Liu L, Corma A. Metal catalysts for heterogeneous catalysis: from single atoms to nanoclusters and nanoparticles. Chemical Reviews, 2018, 118 (10): 4981–5079. DOI: 10.1021/acs.chemrev.7b00776
|
[16] |
Samantaray M K, D’Elia V, Pump E, et al. The comparison between single atom catalysis and surface organometallic catalysis. Chemical Reviews, 2020, 120 (2): 734–813. DOI: 10.1021/acs.chemrev.9b00238
|
[17] |
Lang R, Li T, Matsumura D, et al. Hydroformylation of olefins by a rhodium single-atom catalyst with activity comparable to RhCl(PPh3)3. Angewandte Chemie International Edition, 2016, 55 (52): 16054–16058. DOI: 10.1002/anie.201607885
|
[18] |
Jin H Q, Li P P, Cui P X, et al. Unprecedentedly high activity and selectivity for hydrogenation of nitroarenes with single atomic Co1-N3P1 sites. Nature Communications, 2022, 13 (1): 723. DOI: 10.1038/s41467-022-28367-9
|
[19] |
Wang L L, Wang H W, Lu J L. Local chemical environment effect in single-atom catalysis. Chem Catalysis, 2023, 3 (4): 100492 DOI: 10.1016/j.checat.2022.100492
|
[20] |
Lu B, Liu Q, Chen S. Electrocatalysis of single-atom sites: impacts of atomic coordination. ACS Catalysis, 2020, 10 (14): 7584–7618. DOI: 10.1021/acscatal.0c01950
|
[21] |
Li Y, Xu Y X, Chen S, et al. Tuning the electronic structures of anchor sites to achieve zero‐valence single‐atom catalysts for advanced hydrogenation. Angewandte Chemie International Edition, 2024, 63 (35): e202406262. DOI: 10.1002/anie.202406262
|
[22] |
Li S, Xu Y X, Wang H W, et al. Tuning the CO2 hydrogenation selectivity of rhodium single-atom catalysts on zirconium dioxide with alkali ions. Angewandte Chemie International Edition, 2023, 62 (8): e202218167. DOI: 10.1002/anie.202218167
|
[23] |
Cho J, Lim T, Kim H, et al. Importance of broken geometric symmetry of single-atom Pt sites for efficient electrocatalysis. Nature Communications, 2023, 14: 3233. DOI: 10.1038/s41467-023-38964-x
|
[24] |
Lang R, Du X R, Huang Y K, et al. Single-atom catalysts based on the metal–oxide interaction. Chemical Reviews, 2020, 120 (21): 11986–12043. DOI: 10.1021/acs.chemrev.0c00797
|
[25] |
Wang A Q, Li J, Zhang T. Heterogeneous single-atom catalysis. Nature Reviews Chemistry, 2018, 2: 65–81. DOI: 10.1038/s41570-018-0010-1
|
[26] |
Zheng Y, Wang Q, Yang Q, et al. Boosting the hydroformylation activity of a Rh/CeO2 single-atom catalyst by tuning surface deficiencies. ACS Catalysis, 2023, 13 (11): 7243–7255. DOI: 10.1021/acscatal.3c00810
|
[27] |
Ro I, Qi J, Lee S, et al. Bifunctional hydroformylation on heterogeneous Rh-WO x pair site catalysts. Nature, 2022, 609: 287–292. DOI: 10.1038/s41586-022-05075-4
|
[28] |
Sun Q, Dai Z F, Liu X L, et al. Highly efficient heterogeneous hydroformylation over Rh-metalated porous organic polymers: synergistic effect of high ligand concentration and flexible framework. Journal of the American Chemical Society, 2015, 137 (15): 5204–5209.
|
[29] |
Li C Y, Wang W L, Yan L, et al. A mini review on strategies for heterogenization of rhodium-based hydroformylation catalysts. Frontiers of Chemical Science and Engineering, 2018, 12: 113–123.
|
[30] |
Cao L L, Luo Q Q, Liu W, et al. Identification of single-atom active sites in carbon-based cobalt catalysts during electrocatalytic hydrogen evolution. Nature Catalysis, 2019, 2: 134–141.
|
[31] |
Liu S, Zhu H L, Yao W Q, et al. One step synthesis of P-doped g-C3N4 with the enhanced visible light photocatalytic activity. Applied Surface Science, 2018, 430: 309–315.
|
[32] |
Hu S Z, Ma L, You J G, et al. A simple and efficient method to prepare a phosphorus modified g-C3N4 visible light photocatalyst. RSC Advances, 2014, 4: 21657–21663.
|
[33] |
Ran J, Ma T Y, Gao G, et al. Porous P-doped graphitic carbon nitride nanosheets for synergistically enhanced visible-light photocatalytic H2 production. Energy & Environmental Science, 2015, 8: 3708–3717.
|
[34] |
Ong W J, Tan L L, Ng Y H, et al. Graphitic carbon nitride (g-C3N4)-based photocatalysts for artificial photosynthesis and environmental remediation: Are we a step closer to achieving sustainability. Chemical Reviews, 2016, 116 (12): 7159–7329.
|
[35] |
Yang S, Gong Y, Zhang J, et al. Exfoliated graphitic carbon nitride nanosheets as efficient catalysts for hydrogen evolution under visible light. Advanced Materials, 2013, 25 (17): 2452–2456.
|
[36] |
Pelavin M, Hendrickson D N, Hollander J M, et al. Phosphorus 2p electron binding energies. Correlation with extended Hueckel charges. The Journal of Physical Chemistry, 1970, 74 (5): 1116–1121. DOI: 10.1021/j100700a027
|
[37] |
Zhang Y, Mori T, Ye J, et al. Phosphorus-doped carbon nitride solid: enhanced electrical conductivity and photocurrent generation. Journal of the American Chemical Society, 2010, 132 (18): 6294–6295. DOI: 10.1021/ja101749y
|
[38] |
Ma X G, Lv Y H, Xu J, et al. A strategy of enhancing the photoactivity of g-C3N4 via doping of nonmetal elements: a first-principles study. The Journal of Physical Chemistry C, 2012, 116 (44): 23485–23493. DOI: 10.1021/jp308334x
|
[39] |
Liu B Y, Huang N, Wang Y, et al. Promotion of inorganic phosphorus on Rh catalysts in styrene hydroformylation: geometric and electronic effects. ACS Catalysis, 2021, 11 (3): 1787–1796. DOI: 10.1021/acscatal.0c04684
|
[40] |
Leach M R. Concerning electronegativity as a basic elemental property and why the periodic table is usually represented in its medium form. Foundations of Chemistry, 2013, 15: 13–29.
|
[41] |
Allen L C. Chemistry and electronegativity. International Journal of Quantum Chemistry, 1994, 49 (3): 253–277. DOI: 10.1002/qua.560490315
|
[42] |
Nair V S, Mathew S P, Chaudhari R V. Kinetics of hydroformylation of styrene using homogeneous rhodium complex catalyst. Journal of Molecular Catalysis A: Chemical, 1999, 143: 99–110. DOI: 10.1016/S1381-1169(98)00372-0
|
[43] |
Liu B Y, Huang N, Wang Y, et al. Insights into the activity screening and hydroformylation kinetics of Rh-Based bimetallic phosphides. ACS Catalysis, 2021, 11 (24): 15235–15243. DOI: 10.1021/acscatal.1c03801
|
[44] |
Yu Z N, Zhang S X, Zhang L L, et al. Suppressing metal leaching and sintering in hydroformylation reaction by modulating the coordination of Rh single atoms with reactants. Journal of the American Chemical Society, 2024, 146 (17): 11955–11967. DOI: 10.1021/jacs.4c01315
|