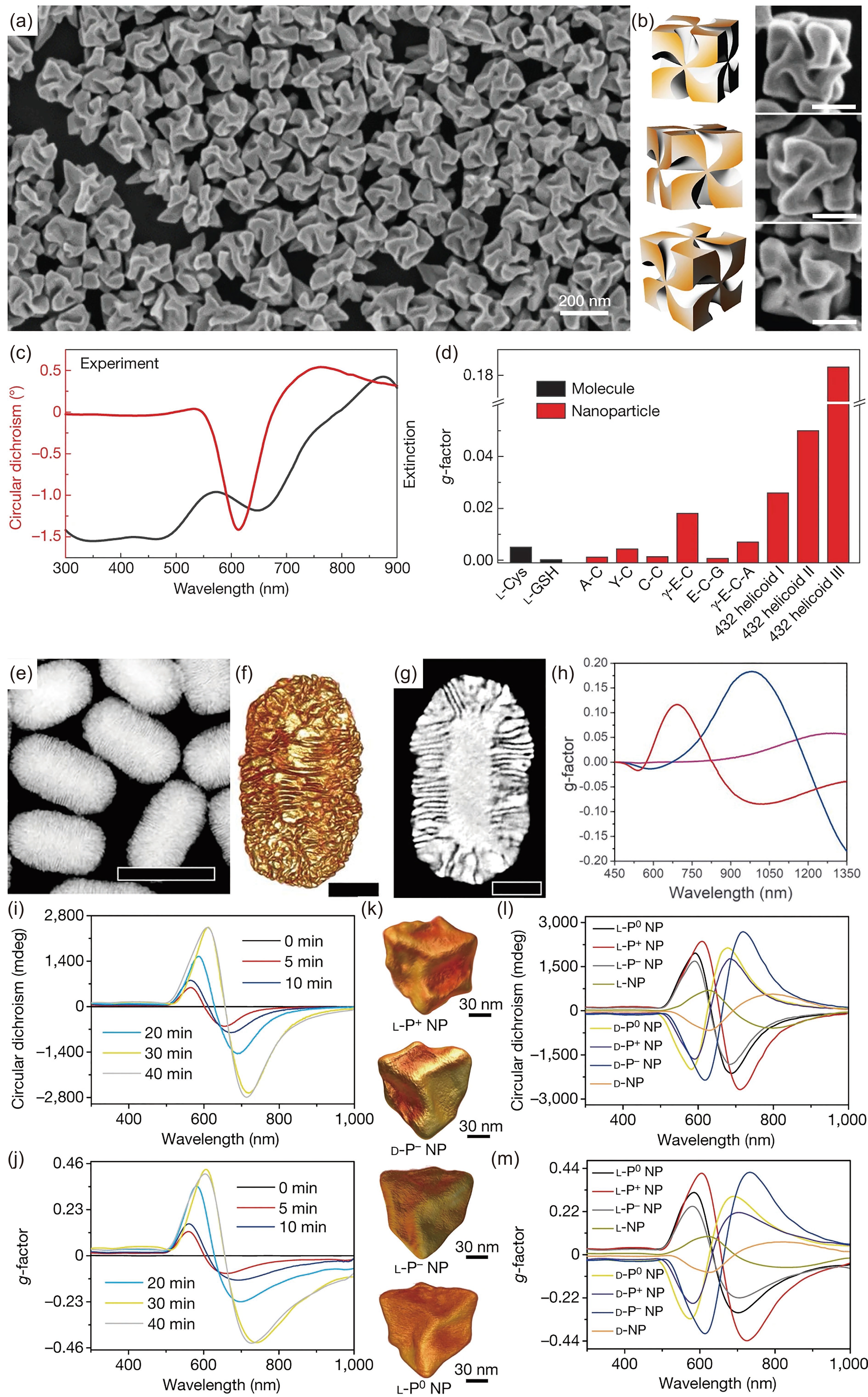
Inorganic chiral nanomaterials have attracted wide attention because of their superior physical properties and chiroptical activities. Great progress in chiral nanostructure preparation has been made, such as noble metals and semiconductors. In this review, we introduce several chiral nanomaterials with feasible biocompatibility and low cytotoxicity that are promising candidates for biological applications, and we focus on their preparation in terms of their circular dichroism (CD) effects and circular luminescence properties. Additionally, we summarize the working function of chiral nanostructures toward some common diseases with high prevalence, such as Alzheimer’s disease (AD), Parkinson’s disease (PD), diabetes and even cancers. The introduction of inorganic chirality will provide a novel way to diagnose and treat these diseases.
Schematic illustration of applications in clinical diseases using chiral inorganic nanostructures.
Figure 1. (a) SEM image, (b) 3D models and corresponding SEM images (scale bars: 100 nm), and (c) experimental CD and extinction spectra of 432 helicoid III chiral NPs induced by L-cysteine. (d) g-factor comparison among 432 helicoid III, other NPs, and chiral molecules. Adapted with permission from Ref. [7]. Copyright 2018, Springer Nature. (e) HAADF-STEM image (scale bars: 200 nm), (f) 3D reconstructions (scale bars: 50 nm) and (g) orthoslices (scale bars: 50 nm) of chiral Au NRs. (h) g-factor spectra of chiral Au NRs with different sizes. Adapted with permission from Ref. [23]. Copyright 2020, the American Association for the Advancement of Science. (i) CD and (j) g-factor spectra of L-P+ NPs exposed to laser irradiation for different durations. (k) TEM tomography images of L-P+, D-P−, L-P−, and L-P0 NPs. (l) CD and (m) g-factor spectra of synthesized chiral Au NPs. Adapted with permission from Ref. [16]. Copyright 2022, Springer Nature.
Figure 2. (a) Structure of DNA-nanocrystal pyramids. (b) Schematic and (c) TEM images of chiral pyramids. Top row, R; bottom row, S. Adapted with permission from Ref. [40]. Copyright 2009, American Chemical Society. (d) Schematic illustration of the Au-UCNP pyramids for microRNA detection. (e) CD and (f) luminescence spectra of Au-UCNP pyramids. Adapted with permission from Ref. [33]. Copyright 2016, American Chemical Society. (g) Tetrahedron NPs centralized with UCNPs. Adapted with permission from Ref. [34]. Copyright 2018, American Chemical Society. (h) Schematic illustration of nanoparticle heterodimer assembly and its application in biological analysis. Adapted with permission from Ref. [41]. Copyright 2013, American Chemical Society. (i) Synthesis of shell core–Au satellite and detection of ochratoxin A based on chiral signal. Adapted with permission from Ref. [39]. Copyright 2018, Wiley-VCH.
Figure 3. (a) CD spectra of L-cysteine-DRs and (b) D-cysteine-DRs. (c) UV/vis spectra of L-cysteine-DRs with different cysteine/DR ratios. (d) Illustration of the relationship between the g-factor of CdSe/Cds DRs and the ratio of cysteine/DRs. (e) CPL spectra and (f) glum values of L- and D-cysteine-DRs with different cysteine/DR ratios. Adapted with permission from Ref. [46]. Copyright 2018, American Chemical Society. (g) glum of chiral CdSe/CdS QDs with different concentrations of cysteine. (h) The relationship between glum and the concentration of cysteine at 605 nm. (i) FTIR spectra of QDs and QDs with cysteine or organic ligands. (j) 1H NMR spectra and (k) 13C NMR spectra of QDs. Adapted with permission from Ref. [47]. Copyright 2019, American Chemical Society. (l) CD spectra of L-/D-pen and the chiral QDs. (m) CD spectra of QDs with various concentrations of chiral Pen. (n) Illustration of the influence of L-QDs on the cleavage of BSA under different light conditions. Lane 1, molecular marker; lane 2, BSA; lane 3, BSA + RCP; lane 4, BSA + LP; lane 5, BSA + LCP. (o) PL spectra of BSA with different concentrations of L-QDs. (p) CD spectra of photolysis of BSA at different reaction times. (q) TEM image of L-QDs after interacting with BSA. (r) XPS Cu 2p spectrum of l-QDs after the reaction. (s) CD spectra of the QDs after the cleavage reaction. Adapted with permission from Ref. [48]. Copyright 2019, Wiley-VCH.
Figure 4. (a) Illustration of the fabrication of chiral GQDs by a three-step approach. (b) UV‒vis and FL spectra of GQDs. Adapted with permission from Ref. [50]. Copyright 2016, the Royal Society of Chemistry. (c) Illustration of chiral CDs as supramolecular templates. (d) UV‒vis spectra of CDs and CDs with HnTSPPm- at different pH values. (e) ECD spectra of L- and D-CDs with HnTSPPm-. Adapted with permission from Ref. [51]. Copyright 2018, Springer Nature. (f) Illustration of the preparation approach for multicolor-emitting chiral CDs. (g) PL spectra of the three types of CDs. (h) CD spectra and (i) CPL of the three CDs capped with gels. (j) Illustration of the CIE value of white PL. (k) glum values of L- and D-CDs. (l) Laser scanning confocal microscope image of white luminescent colloid. Adapted with permission from Ref. [52]. Copyright 2021, Wiley-VCH. (m) PL spectra of the excitation spectrum (black line) and emission spectrum (red line) of L-CDs. (n) Illustration of the relationship of the intensity of the L-CD PL emission. (o) CD spectra of L- and D-Cys and (p) L- and D-CDs. (q–u) Illustration of the influence of chiral CDs on mitochondrial respiration on behalf of the oxygen consumption rate (OCR) of T24 cells treated with 50 µg·mL−1 CDs (q), 100 µg·mL−1 CDs (r), 200 µg·mL−1 CDs (s) for 24 h, (t) basal OCR and (u) maximal OCR. Oligo=oligomycin A, FCCP=carbonyl cyanide-4(trifluoromethoxy)phenylhydrazone, AA=antimycin A, Rot=rotenone. Adapted with permission from Ref. [3]. Copyright 2018, Wiley-VCH.
Figure 5. (a) Representative illustration of enantioselective binding of Aβ by chiral metallosupramolecular complexes. CD spectra demonstrated the efficiency of (b) complex 1 and (c) complex 2 to inhibit Aβ1–40 aggregation. Adapted with permission from Ref. [55]. Copyright 2014, American Chemical Society. (d) Apoptosis pathways of senescent microglial cells in the brains of PD mice induced by chiral NPs. Adapted with permission from Ref. [32]. Copyright 2013, Royal Society of Chemistry. (e) Schematic illustration of the synthesis of chiral Pt@Au TNRs. (f) AFM and (g) 3D tomography images corresponding to L-Pt@Au TNR and D-Pt@Au TNR. (h) CD, (i) g-factor, and (j) simulated CD spectra of chiral Pt@Au TNRs. Adapted with permission from Ref. [22]. Copyright 2021, Wiley-VCH.
Figure 6. (a) Illustration of the acquisition and purification of urine samples and the aggregation of chiral molecules in the urine on MCMs for more sensitive detection by the shift of CD spectra. (b) CD spectra of LH-MCMs and RH-MCMs under interaction with L- and D-glucose during continuous microbubble-assisted accumulation. Adapted with permission from Ref. [65]. Copyright 2021, American Chemical Society. (c) PL spectra (λex = 280 nm) and (d) CD spectra of maltase (black line), maltase/LCDs (blue line), and maltase/DCDs (red line) with maltase and CD concentrations of 2.5 and 1.5 mg/mL, respectively. (e) Illustration of the effect of different hydrolysis times of maltase (black line), maltase/LCDs (blue line), and maltase/DCDs (red line) on the glucose concentration. (f) Illustration of the effect of LCDs and DCDs at different concentrations on the inhibition of maltase. Adapted with permission from Ref. [66]. Copyright 2019, Wiley-VCH.
Figure 7. (a) Illustration of the detection mechanism of chiral Au NPs for AFP. (b) CD and UV‒vis spectra of chiral Au with different concentrations of AFP. (c) Illustration of the logarithmic relationship between CD spectra and the concentration of AFP. Adapted with permission from Ref. [68]. Copyright 2017, Springer Nature. (d) The fabrication process of chiral Au NPs. (e) UV–vis, (f) CD and (g) g-factor spectra of prism and chiral NPs under irradiation at 594 nm with 84 mW· cm−2 for 30 min. (h) The mechanism of action of chiral NPs for tumor therapy. (i) The survival of mice with tumor cells under the influence of PBS, prism, L-NPs and D-NPs. (j) The preventative effects. Adapted with permission from Ref. [34]. Copyright 2022, Wiley-VCH.
[1] |
Lebreton G, Géminard C, Lapraz F, et al. Molecular to organismal chirality is induced by the conserved myosin 1D. Science, 2018, 362 (6417): 949–952. DOI: 10.1126/science.aat8642
|
[2] |
Tang K, Gan H, Li Y, et al. Stereoselective interaction between DNA and chiral surfaces. J. Am. Chem. Soc., 2008, 130 (34): 11284–11285. DOI: 10.1021/ja8044184
|
[3] |
Li F, Li Y, Yang X, et al. Highly fluorescent chiral N-S-doped carbon dots from cysteine: Affecting cellular energy metabolism. Angew. Chem. Int. Ed., 2018, 57 (9): 2377–2382. DOI: 10.1002/anie.201712453
|
[4] |
Qu A, Sun M, Kim J Y, et al. Stimulation of neural stem cell differentiation by circularly polarized light transduced by chiral nanoassemblies. Nat. Biomed. Eng., 2021, 5 (1): 103–113. DOI: 10.1038/s41551-020-00634-4
|
[5] |
Li R S, Gao P F, Zhang H Z, et al. Chiral nanoprobes for targeting and long-term imaging of the Golgi apparatus. Chem. Sci., 2017, 8 (10): 6829–6835. DOI: 10.1039/C7SC01316G
|
[6] |
Wang X, Wang M, Lei R, et al. Chiral surface of nanoparticles determines the orientation of adsorbed transferrin and its interaction with receptors. ACS Nano, 2017, 11 (5): 4606–4616. DOI: 10.1021/acsnano.7b00200
|
[7] |
Lee H E, Ahn H Y, Mun J, et al. Amino-acid- and peptide-directed synthesis of chiral plasmonic gold nanoparticles. Nature, 2018, 556 (7701): 360–365. DOI: 10.1038/s41586-018-0034-1
|
[8] |
Wu C, Yin Y. Chiral semiconductor photonic thin film with tunable circularly polarized luminescence. Matter, 2022, 5 (8): 2466–2468. DOI: 10.1016/j.matt.2022.06.018
|
[9] |
Zheng H, Li W, Li W, et al. Uncovering the circular polarization potential of chiral photonic cellulose films for photonic applications. Adv. Mater., 2018, 30 (13): 1705948. DOI: 10.1002/adma.201705948
|
[10] |
Xu L, Xu Z, Ma W, et al. Highly selective recognition and ultrasensitive quantification of enantiomers. J. Mater. Chem. B, 2013, 1 (35): 4478–4483. DOI: 10.1039/c3tb20692k
|
[11] |
Fan H, Li K, Tu T, et al. ATP-induced emergent circularly polarized luminescence and encryption. Angew. Chem. Int. Ed., 2022, 61 (19): e202200727. DOI: 10.1002/anie.202200727
|
[12] |
Yan J, Yao Y, Yan S, et al. Chiral protein supraparticles for tumor suppression and synergistic immunotherapy: An enabling strategy for bioactive supramolecular chirality construction. Nano Lett., 2020, 20 (8): 5844–5852. DOI: 10.1021/acs.nanolett.0c01757
|
[13] |
Li S, Sun M, Hao C, et al. Chiral Cu xCo yS nanoparticles under magnetic field and NIR light to eliminate senescent cells. Angew. Chem. Int. Ed., 2020, 59 (33): 13915–13922. DOI: 10.1002/anie.202004575
|
[14] |
Miao J, Cai Y, Shao Y N, et al. Multiple cell death pathways triggered by temperature-mediated synergistic effect derived from chiral phototheranostic ablation nanoagents. Appl. Mater. Today, 2021, 23: 101001. DOI: 10.1016/j.apmt.2021.101001
|
[15] |
Ma W, Hao C, Sun M, et al. Tuning of chiral construction, structural diversity, scale transformation and chiroptical applications. Mater. Horiz., 2018, 5 (2): 141–161. DOI: 10.1039/C7MH00966F
|
[16] |
Wang Y, Xia Y. Near-infrared optically active Cu2- xS nanocrystals: Sacrificial template-ligand exchange integration fabrication and chirality dependent autophagy effects. J. Mater. Chem. B, 2020, 8 (35): 7921–7930. DOI: 10.1039/D0TB01223H
|
[17] |
Xu L, Wang X, Wang W, et al. Enantiomer-dependent immunological response to chiral nanoparticles. Nature, 2022, 601 (7893): 366–373. DOI: 10.1038/s41586-021-04243-2
|
[18] |
Zhao X, Xu L, Sun M, et al. Tuning the interactions between chiral plasmonic films and living cells. Nat. Commun., 2017, 8 (1): 2007. DOI: 10.1038/s41467-017-02268-8
|
[19] |
Li Y W, Miao Z W, Shang Z W, et al. A visible- and NIR-light responsive photothermal therapy agent by chirality-dependent MoO3− x nanoparticles. Adv. Funct. Mater., 2020, 30 (4): 1906311. DOI: 10.1002/adfm.201906311
|
[20] |
Wang G, Hao C, Chen C, et al. Six-pointed star chiral cobalt superstructures with strong antibacterial activity. Small, 2022, 18 (39): 2204219. DOI: 10.1002/smll.202204219
|
[21] |
Yeom J, Guimaraes P P G, Ahn H M, et al. Chiral supraparticles for controllable nanomedicine. Adv. Mater., 2020, 32 (1): e1903878. DOI: 10.1002/adma.201903878
|
[22] |
Djunic I, Elezovic I, Marinkovic M, et al. Osteolytic lesions marker in multiple myeloma. Med. Oncol., 2011, 28 (1): 237–240. DOI: 10.1007/s12032-010-9432-4
|
[23] |
Dunn G P, Bruce A T, Ikeda H, et al. Cancer immunoediting: From immunosurveillance to tumor escape. Nat. Immunol., 2002, 3 (11): 991–998. DOI: 10.1038/ni1102-991
|
[24] |
Liu J, Yang L, Qin P, et al. Recent advances in inorganic chiral nanomaterials. Adv. Mater., 2021, 33 (50): 2005506. DOI: 10.1002/adma.202005506
|
[25] |
Wang G, Hao C, Ma W, et al. Chiral plasmonic triangular nanorings with SERS activity for ultrasensitive detection of amyloid proteins in Alzheimer’s disease. Adv. Mater., 2021, 33 (38): 2102337. DOI: 10.1002/adma.202102337
|
[26] |
González-Rubio G, Mosquera J, Kumar V, et al. Micelle-directed chiral seeded growth on anisotropic gold nanocrystals. Science, 2020, 368 (6498): 1472–1477. DOI: 10.1126/science.aba0980
|
[27] |
Han B, Shi L, Gao X, et al. Ultra-stable silica-coated chiral Au-nanorod assemblies: Core–shell nanostructures with enhanced chiroptical properties. Nano Res., 2016, 9 (2): 451–457. DOI: 10.1007/s12274-015-0926-4
|
[28] |
Hao C, Xu L, Sun M, et al. Chirality on hierarchical self-assembly of Au@AuAg yolk-shell nanorods into core-satellite superstructures for biosensing in human cells. Adv. Funct. Mater., 2018, 28 (33): 1802372. DOI: 10.1002/adfm.201802372
|
[29] |
Wang W, Wu F, Zhang Y, et al. Boosting chiral amplification in plasmon-coupled circular dichroism using discrete silver nanorods as amplifiers. Chem. Commun., 2021, 57 (60): 7390–7393. DOI: 10.1039/D1CC01891D
|
[30] |
Wu X, Xu L, Ma W, et al. Propeller-like nanorod-upconversion nanoparticle assemblies with intense chiroptical activity and luminescence enhancement in aqueous phase. Adv. Mater., 2016, 28 (28): 5907–5915. DOI: 10.1002/adma.201601261
|
[31] |
Zheng G, Bao Z, Pérez-Juste J, et al. Tuning the morphology and chiroptical properties of discrete gold nanorods with amino acids. Angew. Chem. Int. Ed., 2018, 57 (50): 16452–16457. DOI: 10.1002/anie.201810693
|
[32] |
Guo X R, Wu D, Li Y, et al. Ordering silver nanowires for chiroptical activity. Sci. China Mater., 2022, 65 (5): 1362–1368. DOI: 10.1007/s40843-021-1899-6
|
[33] |
Tan L, Yu S J, Jin Y, et al. Inorganic chiral hybrid nanostructures for tailored chiroptics and chirality-dependent photocatalysis. Angew. Chem. Int. Ed., 2022, 61 (24): e202112400. DOI: 10.1002/anie.202112400
|
[34] |
Wang W, Zhao J, Hao C, et al. The development of chiral nanoparticles to target NK Cells and CD8+ T cells for cancer immunotherapy. Adv. Mater., 2022, 34 (16): 2109354. DOI: 10.1002/adma.202109354
|
[35] |
Xu Z, Qu A, Zhang H, et al. Photoinduced elimination of senescent microglia cells in vivo by chiral gold nanoparticles. Chem. Sci., 2022, 13 (22): 6642–6654. DOI: 10.1039/D2SC01662A
|
[36] |
Li S, Xu L, Ma W, et al. Dual-mode ultrasensitive quantification of microRNA in living cells by chiroplasmonic nanopyramids self-assembled from gold and upconversion nanoparticles. J. Am. Chem. Soc., 2016, 138 (1): 306–312. DOI: 10.1021/jacs.5b10309
|
[37] |
Sun M, Hao T, Li X, et al. Direct observation of selective autophagy induction in cells and tissues by self-assembled chiral nanodevice. Nat. Commun., 2018, 9 (1): 4494. DOI: 10.1038/s41467-018-06946-z
|
[38] |
Yan W, Xu L, Xu C, et al. Self-assembly of chiral nanoparticle pyramids with strong R/S optical activity. J. Am. Chem. Soc., 2012, 134 (36): 15114–15121. DOI: 10.1021/ja3066336
|
[39] |
Li C, Li S, Zhao J, et al. Ultrasmall magneto-chiral cobalt hydroxide nanoparticles enable dynamic detection of reactive oxygen species in vivo. J. Am. Chem. Soc., 2022, 144 (4): 1580–1588. DOI: 10.1021/jacs.1c09986
|
[40] |
Probst P T, Mayer M, Gupta V, et al. Mechano-tunable chiral metasurfaces via colloidal assembly. Nat. Mater., 2021, 20 (7): 1024–1028. DOI: 10.1038/s41563-021-00991-8
|
[41] |
Yuan A, Hao C, Wu X, et al. Chiral Cu xOS@ZIF-8 nanostructures for ultrasensitive quantification of hydrogen sulfide in vivo. Adv. Mater., 2020, 32 (19): 1906580. DOI: 10.1002/adma.201906580
|
[42] |
Cai J, Hao C, Sun M, et al. Chiral shell core-satellite nanostructures for ultrasensitive detection of mycotoxin. Small, 2018, 14 (13): 1703931. DOI: 10.1002/smll.201703931
|
[43] |
Mastroianni A J, Claridge S A, Alivisatos A P. Pyramidal and chiral groupings of gold nanocrystals assembled using DNA scaffolds. J. Am. Chem. Soc., 2009, 131: 8455–8459. DOI: 10.1021/ja808570g
|
[44] |
Han B, Zhu Z, Li Z, et al. Conformation modulated optical activity enhancement in chiral cysteine and au nanorod assemblies. J. Am. Chem. Soc., 2014, 136 (46): 16104–16107. DOI: 10.1021/ja506790w
|
[45] |
Wu X, Xu L, Liu L, et al. Unexpected chirality of nanoparticle dimers and ultrasensitive chiroplasmonic bioanalysis. J. Am. Chem. Soc., 2013, 135 (49): 18629–18636. DOI: 10.1021/ja4095445
|
[46] |
Zhao X, Xu L, Sun M, et al. Gold-quantum dot core-satellite assemblies for lighting up microRNA in vitro and in vivo. Small, 2016, 12 (34): 4662–4668. DOI: 10.1002/smll.201503629
|
[47] |
Ben-Moshe A, Govorov A O, Markovich G. Enantioselective synthesis of intrinsically chiral mercury sulfide nanocrystals. Angew. Chem. Int. Ed., 2013, 52 (4): 1275–1279. DOI: 10.1002/anie.201207489
|
[48] |
Nakashima T, Kobayashi Y, Kawai T. Optical activity and chiral memory of thiol-capped CdTe nanocrystals. J. Am. Chem. Soc., 2009, 131 (30): 10342–10343. DOI: 10.1021/ja902800f
|
[49] |
Song L, Wang S, Kotov N A, et al. Nonexclusive fluorescent sensing for L/D enantiomers enabled by dynamic nanoparticle-nanorod assemblies. Anal. Chem., 2012, 84 (17): 7330–7335. DOI: 10.1021/ac300437v
|
[50] |
Cheng J, Hao J, Liu H, et al. Optically Active CdSe-dot/CdS-rod nanocrystals with induced chirality and circularly polarized luminescence. ACS Nano, 2018, 12 (6): 5341–5350. DOI: 10.1021/acsnano.8b00112
|
[51] |
Kuznetsova V A, Mates-Torres E, Prochukhan N, et al. Effect of chiral ligand concentration and binding mode on chiroptical activity of CdSe/CdS quantum dots. ACS Nano, 2019, 13 (11): 13560–13572. DOI: 10.1021/acsnano.9b07513
|
[52] |
Hao C, Gao R, Li Y, et al. Chiral semiconductor nanoparticles for protein catalysis and profiling. Angew. Chem. Int. Ed., 2019, 58 (22): 7371–7374. DOI: 10.1002/anie.201902673
|
[53] |
Yang F, Gao G, Wang J, et al. Chiral β-HgS quantum dots: Aqueous synthesis, optical properties and cytocompatibility. J. Colloid Interf. Sci., 2019, 537: 422–430. DOI: 10.1016/j.jcis.2018.11.057
|
[54] |
Ru Y, Ai L, Jia T T, et al. Recent advances in chiral carbonized polymer dots: From synthesis and properties to applications. Nano Today, 2020, 34: 100953. DOI: 10.1016/j.nantod.2020.100953
|
[55] |
Vázquez-Nakagawa M, Rodríguez-Pérez L, Herranz M A, et al. Chirality transfer from graphene quantum dots. Chem. Commun., 2016, 52 (4): 665–668. DOI: 10.1039/C5CC08890A
|
[56] |
Ðorđević L, Arcudi F, D’Urso A, et al. Design principles of chiral carbon nanodots help convey chirality from molecular to nanoscale level. Nat. Commun., 2018, 9 (1): 3442. DOI: 10.1038/s41467-018-05561-2
|
[57] |
Ru Y, Sui L, Song H, et al. Rational design of multicolor-emitting chiral carbonized polymer dots for full-color and white circularly polarized luminescence. Angew. Chem. Int. Ed., 2021, 60 (25): 14091–14099. DOI: 10.1002/anie.202103336
|
[58] |
Ross C A, Poirier M A. Protein aggregation and neurodegenerative disease. Nat. Med., 2004, 10: S10–S17. DOI: 10.1038/nm1066
|
[59] |
Bloem B R, Okun M S, Klein C. Parkinson’s disease. The Lancet, 2021, 397 (10291): 2284–2303. DOI: 10.1016/S0140-6736(21)00218-X
|
[60] |
Li M, Howson S E, Dong K, et al. Chiral metallohelical complexes enantioselectively target amyloid β for treating Alzheimer’s disease. J. Am. Chem. Soc., 2014, 136 (33): 11655–11663. DOI: 10.1021/ja502789e
|
[61] |
Hou K, Zhao J, Wang H, et al. Chiral gold nanoparticles enantioselectively rescue memory deficits in a mouse model of Alzheimer’s disease. Nat. Commun., 2020, 11 (1): 4790. DOI: 10.1038/s41467-020-18525-2
|
[62] |
Kumar J, Eraña H, López-Martínez E, et al. Detection of amyloid fibrils in Parkinson’s disease using plasmonic chirality. Proc. Natl. Acad. Sci. U. S. A., 2018, 115 (13): 3225–3230. DOI: 10.1073/pnas.1721690115
|
[63] |
Malishev R, Arad E, Bhunia S K, et al. Chiral modulation of amyloid beta fibrillation and cytotoxicity by enantiomeric carbon dots. Chem. Commun., 2018, 54 (56): 7762–7765. DOI: 10.1039/C8CC03235A
|
[64] |
Beckman J A, Creager M A. Vascular complications of diabetes. Circ. Res., 2016, 118 (11): 1771–1785. DOI: 10.1161/CIRCRESAHA.115.306884
|
[65] |
The Lancet. Untangling the complications of diabetes. The Lancet, 2018, 391 (10138): 2389. DOI: 10.1016/S0140-6736(18)31317-5
|
[66] |
Pop-Busui R, Januzzi J L, Bruemmer D, et al. Heart failure: An underappreciated complication of diabetes. A consensus report of the american diabetes association. Diabetes Care, 2022, 45 (7): 1670–1690. DOI: 10.2337/dci22-0014
|
[67] |
Bullard K M, Cowie C C, Lessem S E, et al. Prevalence of diagnosed diabetes in adults by diabetes type - United States, 2016. MMWR Morb. Mortal. Wkly. Rep., 2018, 67 (12): 359–361. DOI: http://dx.doi.org/10.15585/mmwr.mm6712a2
|
[68] |
Cole J B, Florez J C. Genetics of diabetes mellitus and diabetes complications. Nat. Rev. Nephrol., 2020, 16 (7): 377–390. DOI: 10.1038/s41581-020-0278-5
|
[69] |
Talasniemi J P, Pennanen S, Savolainen H, et al. Analytical investigation: Assay of D-lactate in diabetic plasma and urine. Clin. Biochem., 2008, 41 (13): 1099–1103. DOI: 10.1016/j.clinbiochem.2008.06.011
|
[70] |
Liu Y, Wu Z, Kollipara P S, et al. Label-free ultrasensitive detection of abnormal chiral metabolites in diabetes. ACS Nano, 2021, 15 (4): 6448–6456. DOI: 10.1021/acsnano.0c08822
|
[71] |
Ngamdee K, Ngeontae W. Circular dichroism glucose biosensor based on chiral cadmium sulfide quantum dots. Sensor. Actuat. B Chem., 2018, 274: 402–411. DOI: 10.1016/j.snb.2018.08.005
|
[72] |
Zhang M, Wang H, Wang B, et al. Maltase decorated by chiral carbon dots with inhibited enzyme activity for glucose level control. Small, 2019, 15 (48): 1901512. DOI: 10.1002/smll.201901512
|
[73] |
Du Z, Guan Y, Ding C, et al. Cross-fibrillation of insulin and amyloid β on chiral surfaces: Chirality affects aggregation kinetics and cytotoxicity. Nano Res., 2018, 11 (8): 4102–4110. DOI: 10.1007/s12274-018-1995-y
|
[74] |
Lin J H, Wei Z J, Mao C M. A label-free immunosensor based on modified mesoporous silica for simultaneous determination of tumor markers. Biosens. Bioelectron., 2011, 29 (1): 40–45. DOI: 10.1016/j.bios.2011.07.063
|
[75] |
Zhao H, Bian S, Yang Y, et al. Chiroplasmonic assemblies of gold nanoparticles as a novel method for sensitive detection of alpha-fetoprotein. Microchim. Acta, 2017, 184 (6): 1855–1862. DOI: 10.1007/s00604-017-2207-2
|
[76] |
Tang L, Li S, Xu L, et al. Chirality-based Au@Ag nanorod dimers sensor for ultrasensitive psa detection. ACS Appl. Mater. Interfaces, 2015, 7 (23): 12708–12712. DOI: 10.1021/acsami.5b01259
|
[77] |
Martin H, Smith L, Tomlinson D. Multidrug-resistant breast cancer: Current perspectives. Breast Cancer:Targets and Therapy, 2014, 6: 1–13. DOI: 10.2147/BCTT.S37638
|
[78] |
Galon J, Bruni D. Tumor immunology and tumor evolution: Intertwined histories. Immunity, 2020, 52 (1): 55–81. DOI: 10.1016/j.immuni.2019.12.018
|
[79] |
Jhunjhunwala S, Hammer C, Delamarre L. Antigen presentation in cancer: Insights into tumour immunogenicity and immune evasion. Nat. Rev. Cancer, 2021, 21 (5): 298–312. DOI: 10.1038/s41568-021-00339-z
|
[80] |
Gao P, Chen S, Liu S, et al. Chiral carbon dots-enzyme nanoreactors with enhanced catalytic activity for cancer therapy. ACS Appl. Mater. Interfaces, 2021, 13 (47): 56456–56464. DOI: 10.1021/acsami.1c16091
|