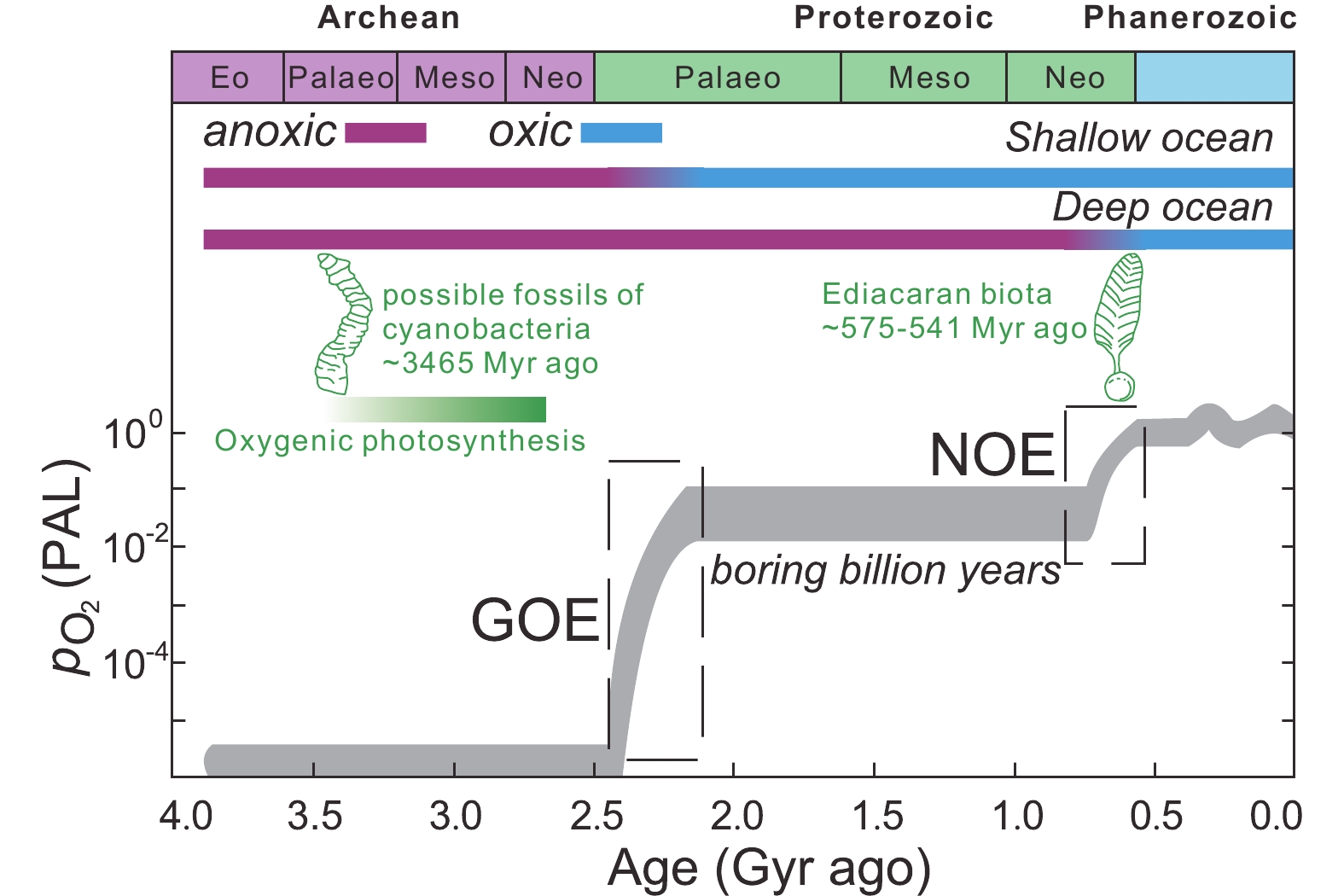
The evolution of the atmospheric oxygen content through Earth’s history is a key issue in paleoclimatic and paleoenvironmental research. There were at least two oxygenation events in the Precambrian that involved fundamental changes in both biotic innovation and the surface environment. However, a large dissolved organic carbon (DOC) pool maintained in deep oceans during the Neoproterozoic may have extended the time interval between the two oxygenation events. To test the DOC hypothesis, we conducted detailed micro-drilled analyses of carbonate carbon isotopes (δ13Ccarb) of a long Ediacaran drill core (the Wangji drill core), for which whole-rock δ13Ccarb and organic carbon isotope (δ13Corg) records were available. The micro-drilled δ13Ccarb values obtained in this study are consistent with whole-rock δ13Ccarb results, precluding the influence of severe authigenic carbonate incorporation. Importantly, the multiple negative δ13Ccarb excursions in the Wangji drill core were likely linked with upwelling events, during which DOC was supplied to the surface water and oxidized. Using box models, we estimate that ~3.6 × 1019 mol and ~2.0 × 1019 mol DOC were converted to bicarbonate during two negative δ13Ccarb excursions spanning millions of years. The estimations are approximately 1000 times the modern marine DOC reservoir. Our results support a relatively high oxidation capacity (elevated atmospheric pO2 and/or oceanic [
Carbon species conversions between dissolved inorganic carbon (DIC) and dissolved organic carbon (DOC) in the Ediacaran ocean.
Figure 2. Schematic diagrams of two dominant primary producers regulating the marine organic carbon cycle, modified after Butterfield[17]. (a) Cyanobacteria-dominated oceans. (b) Algae-dominated oceans. DOC, dissolved organic carbon.
Figure 3. (a) Generalized geological map of China. (b) Paleogeographic reconstruction of the Yangtze platform (South China) consisting of different facies associations during the Ediacaran, modified after Jiang et al.[32]. The red star marks the location of the Wangji drill core. (c) Transect from north to south in the Yangtze platform and the location of the Wangji drill core. (d) Lithostratigraphic, whole-rock δ13Ccarb, and δ13Corg profiles of the Wangji drill core. The pink shading represents phosphorite-rich intervals. The ages are based on U/Pb dating by Condon et al.[34] and Chen et al.[35]. (E) Cross-plot of the whole-rock δ13Ccarb and δ13Corg values of the Wangji drill core.
Figure 9. δ13Corg, δ13Ccarb, Δ13Ccarb-org, total organic carbon content (TOC), and δ18Ocarb profiles for the Wangji drill core. Yellow points represent micro-drilled δ13Ccarb and δ18Ocarb results obtained in this study. The pink shading represents phosphorite-rich intervals. The ages are based on U/Pb dating by Condon et al.[34] and Chen et al.[35].
Figure
10.
Simple reservoir model of oceanic C cycling. Ms is the mass of the surface ocean DIC with the C isotope composition of δs; Md is the mass of the deep ocean DIC with the C isotope composition of δd; MDOC represents the mass of the deep ocean DOC with the C isotope composition of δDOC; Fi represents inputs of carbon from continental weathering, with an average isotopic composition of δi; Fsd is the inorganic carbon flux from the surface to the deep ocean;
Figure 11. Comparison of the observed (three-point average) and modeled C isotope compositions in phosphorite-rich units in the Doushantuo Formation and model predictions of FROC and Δm/Ms* (the mass of remineralized DIC normalized by the initial DIC mass of the surface ocean). Time (t) is estimated by the deposition rate.
[1] |
Farquhar J, Bao H, Thiemens M. Atmospheric influence of Earth’s earliest sulfur cycle. Science, 2000, 289 (5480): 756–758. DOI: 10.1126/science.289.5480.756
|
[2] |
Fike D A, Grotzinger J P, Pratt L M, et al. Oxidation of the Ediacaran ocean. Nature, 2006, 444 (7120): 744–747. DOI: 10.1038/nature05345
|
[3] |
Holland H D. The oxygenation of the atmosphere and oceans. Phil Trans R Soc B, 2006, 361 (1470): 903–915. DOI: 10.1098/rstb.2006.1838
|
[4] |
Frei R, Gaucher C, Poulton S W, et al. Fluctuations in Precambrian atmospheric oxygenation recorded by chromium isotopes. Nature, 2009, 461 (7261): 250–253. DOI: 10.1038/nature08266
|
[5] |
Och L M, Shields-Zhou G A. The Neoproterozoic oxygenation event: Environmental perturbations and biogeochemical cycling. Earth-Science Reviews, 2012, 110 (1–4): 26–57. DOI: 10.1016/j.earscirev.2011.09.004
|
[6] |
Sahoo S K, Planavsky N J, Kendall B, et al. Ocean oxygenation in the wake of the Marinoan glaciation. Nature, 2012, 489 (7417): 546–549. DOI: 10.1038/nature11445
|
[7] |
Lyons T W, Reinhard C T, Planavsky N J. The rise of oxygen in Earth’s early ocean and atmosphere. Nature, 2014, 506 (7488): 307–315. DOI: 10.1038/nature13068
|
[8] |
Luo G, Ono S, Beukes N J, et al. Rapid oxygenation of Earths atmosphere 2.33 billion years ago. Science Advances, 2016, 2 (5): e1600134–e1600134. DOI: 10.1126/sciadv.1600134
|
[9] |
Gumsley A P, Chamberlain K R, Bleeker W, et al. Timing and tempo of the Great Oxidation Event. Proc Natl Acad Sci USA, 2017, 114 (8): 1811–1816. DOI: 10.1073/pnas.1608824114
|
[10] |
Pavlov A A, Kasting J F. Mass-independent fractionation of sulfur isotopes in Archean sediments: Strong evidence for an anoxic Archean atmosphere. Astrobiology, 2002, 2 (1): 27–41. DOI: 10.1089/153110702753621321
|
[11] |
Farquhar J, Wing B A. Multiple sulfur isotopes and the evolution of the atmosphere. Earth and Planetary Science Letters, 2003, 213: 1–13. DOI: 10.1016/S0012-821X(03)00296-6
|
[12] |
Hardisty D S, Lu Z, Planavsky N J, et al. An iodine record of Paleoproterozoic surface ocean oxygenation. Geology, 2014, 42 (7): 619–622. DOI: 10.1130/G35439.1
|
[13] |
McFadden K A, Huang J, Chu X, et al. Pulsed oxidation and biological evolution in the Ediacaran Doushantuo Formation. Proc Natl Acad Sci USA, 2008, 105 (9): 3197–3202. DOI: 10.1073/pnas.0708336105
|
[14] |
Papineau D. Global biogeochemical changes at both ends of the proterozoic: Insights from phosphorites. Astrobiology, 2010, 10 (2): 165–181. DOI: 10.1089/ast.2009.0360
|
[15] |
Planavsky N J, Rouxel O J, Bekker A, et al. The evolution of the marine phosphate reservoir. Nature, 2010, 467 (7319): 1088–1090. DOI: 10.1038/nature09485
|
[16] |
Brocks J J, Jarrett A J M, Sirantoine E, et al. The rise of algae in Cryogenian oceans and the emergence of animals. Nature, 2017, 548 (7669): 578–581. DOI: 10.1038/nature23457
|
[17] |
Butterfield N J. Oxygen, animals and oceanic ventilation: An alternative view. Geobiology, 2009, 7 (1): 1–7. DOI: 10.1111/j.1472-4669.2009.00188.x
|
[18] |
Rothman D H, Hayes J M, Summons R E. Dynamics of the Neoproterozoic carbon cycle. Proc Natl Acad Sci USA, 2003, 100 (14): 8124–8129. DOI: 10.1073/pnas.0832439100
|
[19] |
Hoefs J. Stable Isotope Geochemistry. Sixth edition. Berlin: Springer-Verlag, 2009: 48-53.
|
[20] |
Kump L R, Arthur M A. Interpreting carbon-isotope excursions: Carbonates and organic matter. Chemical Geology, 1999, 161 (1–3): 181–198. DOI: 10.1016/S0009-2541(99)00086-8
|
[21] |
Hayes J M, Strauss H, Kaufman A J. The abundance of 13C in marine organic matter and isotopic fractionation in the global biogeochemical cycle of carbon during the past 800 Ma. Chemical Geology, 1999, 161 (1-3): 103–125. DOI: 10.1016/S0009-2541(99)00083-2
|
[22] |
Halverson G P, Hoffman P F, Schrag D P, et al. Toward a Neoproterozoic composite carbon-isotope record. Geological Society of America Bulletin, 2005, 117 (9–10): 1181–1207. DOI: 10.1130/B25630.1
|
[23] |
Swanson-Hysell N L, Rose C V, Calmet C C, et al. Cryogenian glaciation and the onset of carbon-isotope decoupling. Science, 2010, 328 (5978): 608. DOI: 10.1126/science.1184508
|
[24] |
Schrag D P, Higgins J A, Macdonald F A, et al. Authigenic carbonate and the history of the global carbon cycle. Science, 2013, 339 (6119): 540–543. DOI: 10.1126/science.1229578
|
[25] |
Sun X, Turchyn A V. Significant contribution of authigenic carbonate to marine carbon burial. Nature Geoscience, 2014, 7 (3): 201–204. DOI: 10.1038/ngeo2070
|
[26] |
Cui H, Xiao S, Zhou C, et al. Phosphogenesis associated with the Shuram Excursion: Petrographic and geochemical observations from the Ediacaran Doushantuo Formation of South China. Sedimentary Geology, 2016, 341: 134–146. DOI: 10.1016/j.sedgeo.2016.05.008
|
[27] |
Cui H, Kaufman A J, Xiao S, et al. Was the Ediacaran Shuram Excursion a globally synchronized early diagenetic event? Insights from methane-derived authigenic carbonates in the uppermost Doushantuo Formation, South China. Chemical Geology, 2017, 450: 59–80. DOI: 10.1016/j.chemgeo.2016.12.010
|
[28] |
Gao Y, Zhang X L, Zhang G J, et al. Ediacaran negative C-isotopic excursions associated with phosphogenic events: Evidence from South China. Precambrian Research, 2018, 307: 218–228. DOI: 10.1016/j.precamres.2018.01.014
|
[29] |
Gao Y, Zhang X, Xu Y, et al. High primary productivity during the Ediacaran Period revealed by the covariation of paired C-isotopic records from South China. Precambrian Research, 2020, 349: 105411. DOI: 10.1016/j.precamres.2019.105411
|
[30] |
Li Z X, Li X H, Kinny P D, et al. The breakup of Rodinia: Did it start with a mantle plume beneath South China? Earth and Planetary Science Letters, 1999, 173 (3): 171–181. DOI: 10.1016/S0012-821X(99)00240-X
|
[31] |
Wang J, Li Z. History of Neoproterozoic rift basins in South China: implications for Rodinia break-up. Precambrian Research, 2003, 122 (1–4): 141–158. DOI: 10.1016/S0301-9268(02)00209-7
|
[32] |
Jiang G, Shi X, Zhang S, et al. Stratigraphy and paleogeography of the Ediacaran Doushantuo Formation (ca. 635–551 Ma) in South China. Gondwana Research, 2011, 19 (4): 831–849. DOI: 10.1016/j.gr.2011.01.006
|
[33] |
Gao Y, Zhang X, Fang C, et al. Reconstruction of the Ediacaran sulfur cycle and oceanic redox evolution in shallow-water regions of the Yangtze platform, South China. Precambrian Research, 2021, 353: 106004. DOI: 10.1016/j.precamres.2020.106004
|
[34] |
Condon D, Zhu M, Bowring S, et al. U-Pb ages from the Neoproterozoic Doushantuo Formation, China. Science, 2005, 308 (5718): 95–98. DOI: 10.1126/science.1107765
|
[35] |
Chen D, Zhou X, Fu Y, et al. New U-Pb zircon ages of the Ediacaran-Cambrian boundary strata in South China. Terra Nova, 2015, 27 (1): 62–68. DOI: 10.1111/ter.12134
|
[36] |
Jiang G, Kaufman A J, Christie-Blick N, et al. Carbon isotope variability across the Ediacaran Yangtze platform in South China: Implications for a large surface-to-deep ocean δ13C gradient. Earth and Planetary Science Letters, 2007, 261 (1–2): 303–320. DOI: 10.1016/j.jpgl.2007.07.009
|
[37] |
Zhou C, Xiao S. Ediacaran δ13C chemostratigraphy of South China. Chemical Geology, 2007, 237 (1–2): 89–108. DOI: 10.1016/j.chemgeo.2006.06.021
|
[38] |
Zhu M, Lu M, Zhang J, et al. Carbon isotope chemostratigraphy and sedimentary facies evolution of the Ediacaran Doushantuo Formation in western Hubei, South China. Precambrian Research, 2013, 225: 7–28. DOI: 10.1016/j.precamres.2011.07.019
|
[39] |
Wang X, Jiang G, Shi X, et al. Paired carbonate and organic carbon isotope variations of the Ediacaran Doushantuo Formation from an upper slope section at Siduping, South China. Precambrian Research, 2016, 273: 53–66. DOI: 10.1016/j.precamres.2015.12.010
|
[40] |
Nichols G. Sedimentology and Stratigraphy. Second edition. Chichester, West Sussex, UK: Wiley-Blackwell, 2009: 28-43.
|
[41] |
Kump L R. Interpreting carbon-isotope excursions: Strangelove oceans. Geology, 1991, 19 (4): 299–302. DOI: 10.1130/0091-7613(1991)019<0299:ICIESO>2.3.CO;2
|
[42] |
Zhang G, Zhang X, Shen Y. Quantitative constraints on carbon cycling and temporal changes in episodic euxinia during the end-Permian mass extinction in South China. Chemical Geology, 2021, 562: 120036. DOI: 10.1016/j.chemgeo.2020.120036
|
[43] |
Shi W, Li C, Algeo T J. Quantitative model evaluation of organic carbon oxidation hypotheses for the Ediacaran Shuram carbon isotopic excursion. Science China Earth Sciences, 2017, 60 (12): 2118–2127. DOI: 10.1007/s11430-017-9137-1
|
[44] |
Gill B C, Lyons T W, Young S A, et al. Geochemical evidence for widespread euxinia in the Later Cambrian ocean. Nature, 2011, 469 (7328): 80–83. DOI: 10.1038/nature09700
|
[45] |
Fakhraee M, Tarhan L G, Planavsky N J, et al. A largely invariant marine dissolved organic carbon reservoir across Earth’s history. Proc Natl Acad Sci USA, 2021, 118 (40): e2103511118. DOI: 10.1073/pnas.2103511118
|
[46] |
Bristow T F, Kennedy M J. Carbon isotope excursions and the oxidant budget of the Ediacaran atmosphere and ocean. Geology, 2008, 36: 863–866. DOI: 10.1130/G24968A.1
|
[47] |
Jiang G, Wang X, Shi X, et al. Organic carbon isotope constraints on the dissolved organic carbon (DOC) reservoir at the Cryogenian–Ediacaran transition. Earth and Planetary Science Letters, 2010, 299: 159–168. DOI: 10.1016/j.jpgl.2010.08.031
|
[48] |
Shields G A, Mills B J W, Zhu M, et al. Unique Neoproterozoic carbon isotope excursions sustained by coupled evaporite dissolution and pyrite burial. Nature Geoscience, 2019, 12: 823–827. DOI: 10.1038/s41561-019-0434-3
|